Scientific description of Early Blight Disease (2020)
Based on Adolf B. et al. (2020) Fungal, Oomycete, and Plasmodiophorid Diseases of Potato. In: Campos H., Ortiz O. (eds) The Potato Crop. Springer, Cham
The authors of this content are Hans Hausladen and Birgit Adolf
Symptoms
Foliar symptoms of early blight (EB) are dark brown to black necrosis. First foliar symptoms become visible on the lowest and therefore oldest leaves just a few weeks after emergence. Initially, dark brown dot-like blotches appear, a few millimeters in diameter. The necrotic area gradually increases and the leaf symptoms grow to take up the whole of the green leaf tissue (image below).
Often, the lesions are restricted by leaf veins and take on an angular shape. The size of the necrosis can vary in width, from a few millimeters to 2 cm. Within larger lesions, a series of dark concentric rings are visible.
This target pattern is typical of EB symptoms. Subsequently, the necrotic leaf tissue is often surrounded by a chlorotic border caused by fungal mycotoxins, which turn the leaf tissue yellow. The chlorosis can extend to the whole infected leaf. During EB progression the infected areas enlarge, and the whole leaf becomes necrotic and falls off.
In Europe, a heavy increase of EB infestation occurs from mid-July onwards, especially during hot and dry weather or when the potato crop is under stress, and on physiologically older plants. EB then starts spreading from the lower leaves to the middle and finally upper leaf levels.
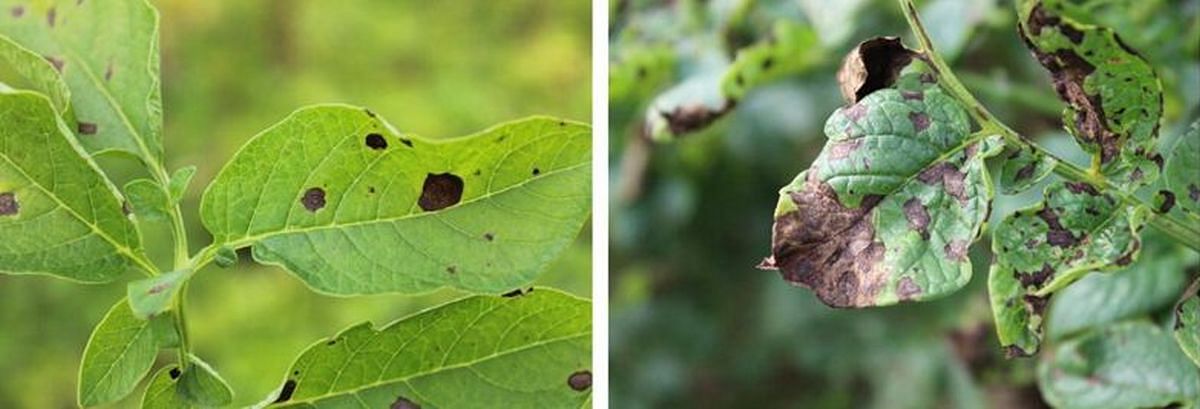
Initial (left) and advanced (right) symptoms of potato early blight in foliage
Alternaria conidia which are washed of the leaves can also infect tubers. The symptoms of EB on tubers are dark, slightly sunken lesions (image below). The dry or hard rot of tubers causes storage losses, reduces quality of table potatoes, and reduces germination capacity of seed potatoes. Open image in new window.
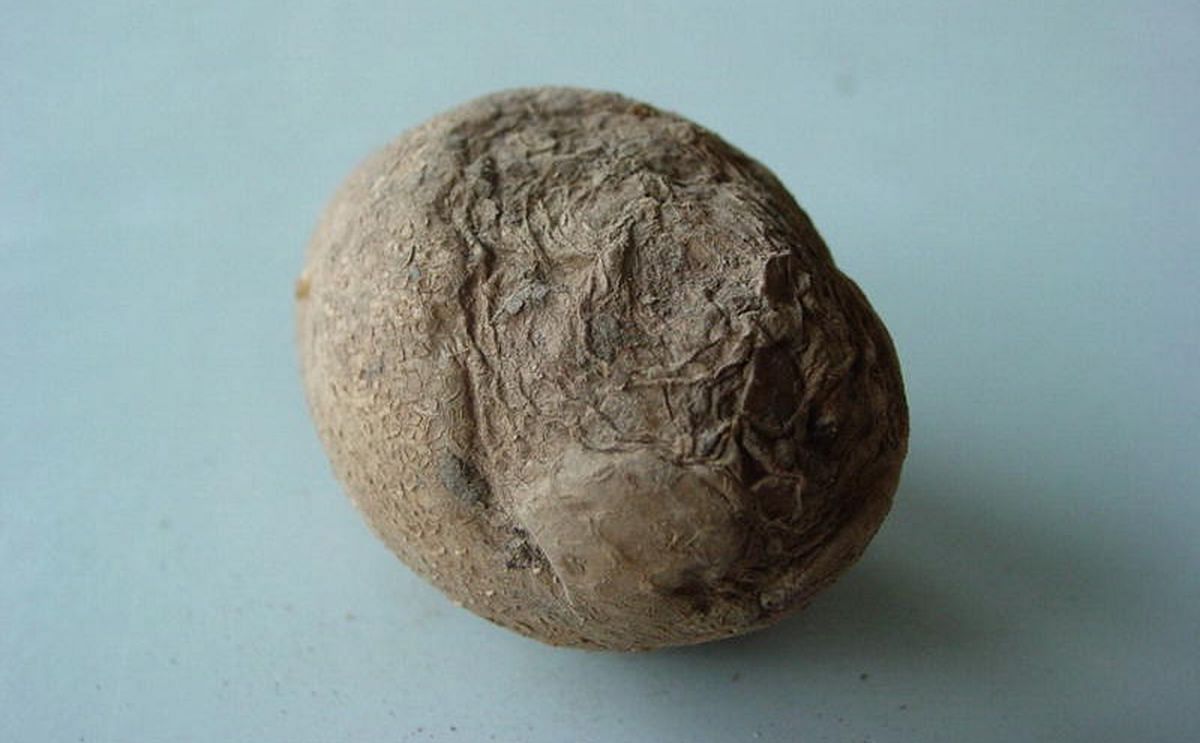
Symptoms of potato early blight in tubers
It isn’t possible to distinguish between the different Alternaria spp. causing EB based on the symptoms even though sometimes symptoms referred to as brown leaf spot (small, irregular to circular, dark brown spots ranging in size from a pinpoint to 4 mm) are attributed to A. alternata (Fairchild et al. 2013).
Causal Organism
The main causal agent of early blight on solanaceous crops is generally considered to be Alternaria solani Sorauer (Gannibal et al. 2014). However, there are reports of other large-spored Alternaria spp. involved in EB of potato.
Rodrigues et al. (2010) found that A. grandis Simmons, but not A. solani, was the causal agent of EB affecting potato plants in several growing regions in Brazil, and Duarte et al. (2014) confirmed that this species can cause EB on potato in field trials using artificial inoculation with A. grandis.
Alternaria protenta has been detected as the causal Alternaria spp. for EB in Algeria (Ayad et al. 2017) and was, together with A. grandis and A. solani, found to be part of the complex of Alternaria spp. detected on EB lesions in Belgium (Landschoot et al. 2017a).
Of the small-spored Alternaria spp., A. alternata occurs on EB lesions on a regular basis, but is considered as a secondary invader (Leiminger et al. 2014; Stammler et al. 2014; Rotem 1994).
Alternaria solani overwinters as mycelium, chlamydiospores or conidia in the soil and infested plant debris (see disease cycle in image below). In spring, the primary infection occurs through inoculum (conidia) carried to the lower leaves by rain splashes. The pathogen is able to penetrate the leaf tissue directly through the intact epidermis or through stomata and wounds.
First symptoms of EB on leaves become visible 2–4 weeks after the emergence of the potato crop. Initially, the older leaves closer to the ground are infested. The fungus is restricted to the lower leaf level for several weeks and seems initially to be of no concern.
Conidia formation occurs on the necrotic leaf tissue at temperatures between 5 and 30 °C (optimum 20 °C). This secondary inoculum is disseminated by wind and causes infections of the surrounding leaves and stems. The latency period averages 3–7 days. During favorable infection conditions, and once the plant has got to a certain age, A. solani very rapidly colonizes the middle and upper leaf layers.
In the field, a cascade-like progression of the fungus from the lower, via the middle, to the upper leaves is visible. Heavily infested leaves fall off and remain as inoculum source on and in the soil.
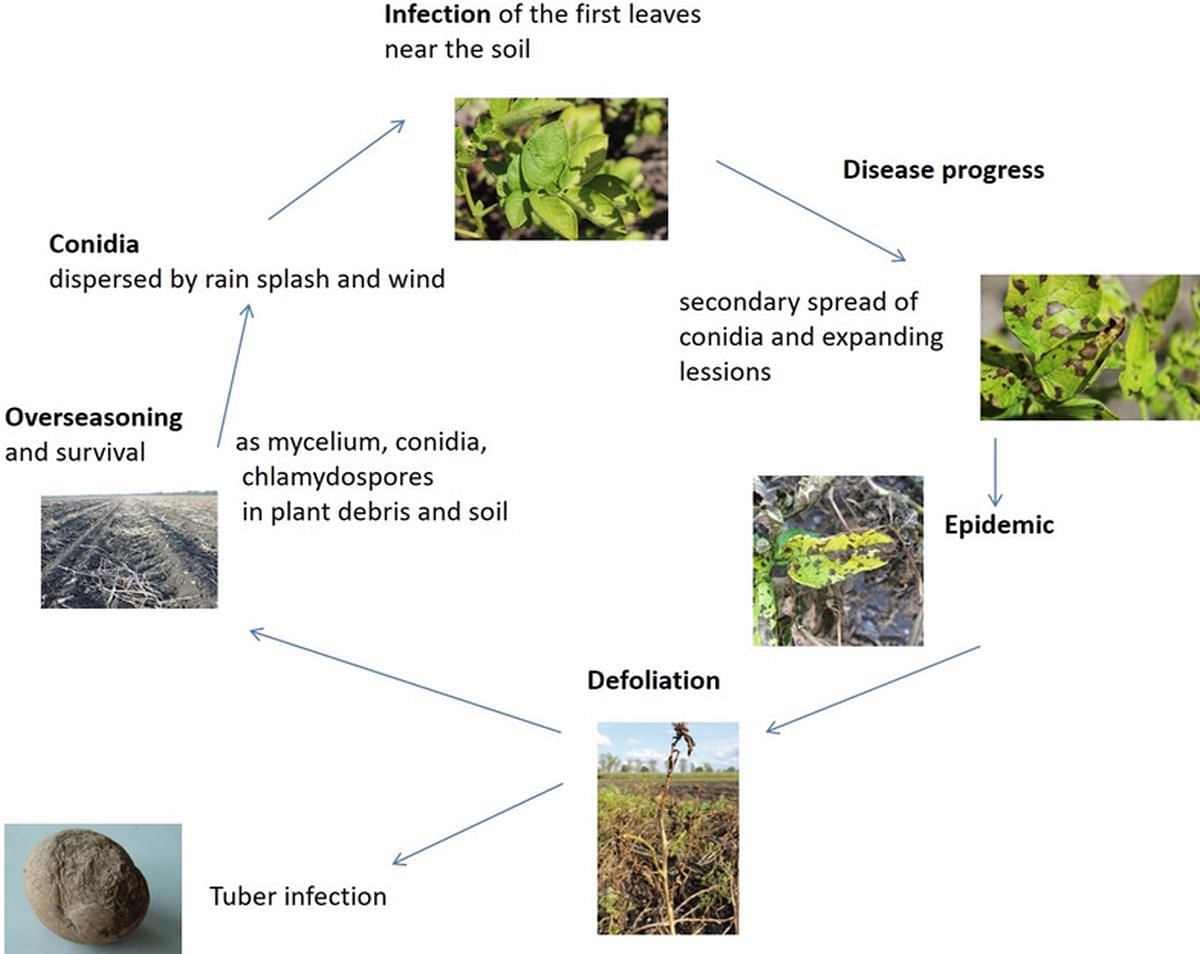
Disease cycle of potato early blight
The disease progression of EB depends on weather parameters, plant age/crop growth stage, maturity group, susceptibility of the cultivar and inoculum concentration, the latter being influenced by short crop rotations.
Favorable weather conditions for infection with A. solani are temperatures above 22 °C and leaf wetness for more than 8 h. Furthermore, alternating wet and dry periods favor disease development.
An important factor for disease progression is the crop growth stage. In addition to the cascade-like spread within plants upwards from older to younger leaves, older plants are significantly more susceptible due to the earlier planting emergence time.
Tuber infestation is caused by conidia that are washed into the soil. The fungus can enter the tuber tissue through lenticels or mechanical injuries of the skin during harvest.
EB can also occur on other host plants apart from potatoes. It has been observed on hosts such as tomato (Solanum lycopersicum L.), eggplants (S. melongena L.), hairy nightshade (S. sarrachoides Sendt), black nightshade (S. nigrum L), horse nettle (S. carolinense L.), pepper (Capsicum spp.), and non-solanaceous weeds (Jones et al. 1993; Pscheidt 1985; Hausladen and Aselmeyer 2017).
Differentiation of Alternaria species within the large-spored or the small-spored group based on morphological traits is time-consuming and requires experience. The molecular approach to delineate large-spored isolates fast and precise is a multi-locus analysis based on different partial gene regions, like the internal transcribed spacer regions 1 and 2 and intervening 5.8S nrDNA (ITS), glyceraldehyde-3-phosphate dehydrogenase (GAPDH), RNA polymerase second largest subunit (RPB2), translation elongation factor 1-alpha (TEF1), the Alternaria major allergen gene (Alt a 1) (Woudenberg et al. 2014).
Based on the RPB2 and calmodulin gene sequence A. solani can be distinguished from A. grandis and A. protenta (Landschoot et al. 2017a). For the small-spored species the glyceraldehyde-3-phosphate dehydrogenase (gapdh), RNA polymerase second largest subunit (rpb2), translation elongation factor 1-alpha (tef1), Alternaria major allergen gene (Alt a 1), endopolygalacturonase (endoPG), an anonymous gene region (OPA10-2), and the histone H3 gene can be used for differentiation (Woudenberg et al. 2015; Landschoot et al. 2017a).
Impact of Early Blight
After potato late blight (Phytophthora infestans), EB represents one of the most important fungal diseases of potato today. It can be found in most potato-growing countries (Woudenberg et al. 2014), but A. solani is described as a very important pathogen especially in warmer areas due to the requirement for higher temperatures.
In susceptible potato cultivars in particular, as well as in locations with increased occurrence of A. solani, the disease can cause considerable yield losses. The potential EB-induced damage for different countries is shown in Table below.
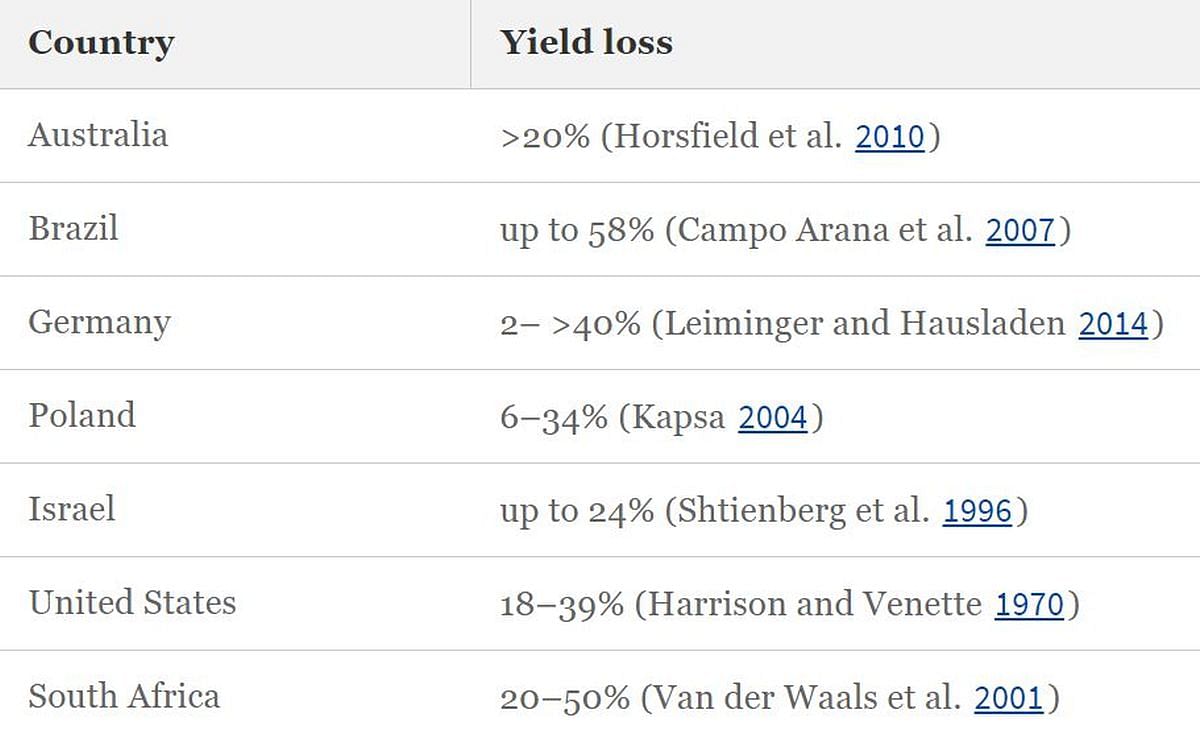
Yield loss due to early blight in different regions.
The necrosis of leaf tissue and considerable defoliation caused by Alternaria infestation reduces the assimilation area of the potato plant, and therefore has a negative effect on tuber size and starch content. Alternaria solani is also able to infect tubers.
The subsequent hard rot of the tubers leads to reduced quality for marketing as table and processing potatoes. In Europe, the occurrence of tuber infections is known only in exceptional years.
Pathogen Populations
The genetic diversity among A. solani isolates is relatively high for an asexually reproducing fungus. Furthermore, when analyzed by virulence assays (VC), random amplified microsatellites (RAMS), random amplified polymorphic DNA (RAPD), and AFLP marker techniques, there is no clear clustering of isolates according to geographical origin, year, or even deriving from the same field (Van der Waals et al. 2004; Leiminger et al. 2013; Odilbekov et al. 2016).
Polymorphism exists even within the usually strongly conserved mitochondrial DNA. Concerning the cytb gene, Leiminger et al. (2014) detected two genotypes in German A. solani populations which differed in their intron–exon structure.
The occurrence of the two genotypes was confirmed for A. solani populations in the USA (Bauske et al. 2018), Belgium (Landschoot et al. 2017b), and Sweden (Odilbekov et al. 2016).
The registration of Alternaria-specific fungicides with single site modes of action for potato changed the population structure of A. solani in many countries due to the occurrence of point mutations, leading to reduced sensitivity of mutated isolates.
For Quinone outside inhibitor fungicides (QoIs) like Azoxystrobin, the change of amino acid phenylalanine to leucine at position 129 in the cytb gene (F129L) has been demonstrated to be the reason for reduced sensitivity compared to wild-type isolates (Pasche et al. 2005).
The presence of the F129L mutation in A. solani populations has been shown in different countries such as the USA (Pasche et al. 2004), Germany (Leiminger et al. 2014), Sweden (Odilbekov et al. 2016), and Belgium (Landschoot et al. 2017b).
The loss in sensitivity towards succinate dehydrogenase inhibitor fungicides (SDHIs) like Boscalid can be caused by different point mutations, as the SDH is composed of four subunits and the binding site of the fungicides is formed by three of them (subunits B, C, D).
For A. solani populations, five possible mutations have been described so far: H278Y and H278R in subunit B, H134R and H134Q in subunit C, and D123R and H133R in subunit D (Mallik et al. 2014; Metz et al. 2019).
Landschoot et al. (2017b) describe the presence of two different SDH mutations in one isolate. Frequently, isolates carry both the F129L mutation in the cytb gene and one of the SDH mutations (Landschoot et al. 2017b).
The sensitivity of baseline isolates to demethylation inhibiting fungicides (DMIs) can vary substantially, but nonbaseline isolates remain sensitive, whereas a distinct loss in sensitivity to anilino-pyrimidine (AP) fungicide pyrimethanil exists.
The primary mode of action has not yet been discovered for AP chemistries; therefore the resistance mechanism is currently unknown for this established fungicide group (Fonseka and Gudmestad 2016).
Resistance against QoIs in A. alternata populations is caused by the G143A mutation in the cytb gene (Ma et al. 2003). Mutations conferring reduced sensitivity to SDHIs for this Alternaria species are the H277R/Y in SDH subunit B, H134R in subunit C, and D123R and H133R in subunit D (Avenot et al. 2008, 2009). Fairchild et al. (2013) detected isolates resistant to pyrimethanil and Fonseka and Gudmestad (2016) stated high DMI EC50 values in baseline isolates.
Management
Integrated pest management to control early blight requires the implementation of several approaches. The disease is primarily controlled by the use of cultural practices (to reduce the soil born inoculum), less susceptible cultivars and the use of pesticides.
Phytosanitary aspects
One of the main components in this case is the crop rotation, which influences the occurrence of early blight. The fungus A. solani persists as mycelium or spores in plant debris or soil in the field from one potato-growing season to the next.
Therefore crop rotation, including the control of host plants such as weeds (black shadow) in the nonhost crops, reduces the initial soil born inoculum.
A short crop rotation with host crops (tomato, potato) results in an earlier and more severe early blight epidemic (Shtienberg and Fry 1990). In addition, the removal or burning of infected plant debris reduces the inoculum level.
A further option to reduce the primary inoculum in the soil is biofumigation. Biofumigation means the suppression of soilborne pathogens by isothiocyanates (ITCs), which derive from hydrolization of glucosinolates by myrosinase in disrupted plant cells. Biofumigant plants (e.g. white mustard, leaf radish) can reduce the early blight disease progression in the crop (Volz et al. 2013).
Tuber harvest and storage
Alternaria solani also can infect the potato tuber. The fungus cannot infect through the intact periderm, and so the risk of tuber infection can be reduced by allowing tubers to fully mature before harvest. Avoiding wounding at harvest and providing storage conditions to promote wound healing can also reduce tuber infection (Venette and Harrison 1973).
Pathogen-free seed
The use of disease- and virus-free seed potatoes is the basis for an economical potato production. Virus-infected potato plants are more susceptible to early blight than healthy plants.
Biotic and abiotic stress
Potato plants stressed by biotic or abiotic factors are more susceptible to early blight disease compared to nonstressed plants. There are different types of abiotic stress for plants during the growing season, such as drought, high temperature, and overhead irrigation.
Additionally, overhead irrigation can prolong the leaf wetness period, allowing successful fungal infection. Biotic stress is driven by insects (e.g. aphids, Colorado beetle), which are also known to be virus vectors.
Plant nutrition
A balanced nutrition for the potato plants during the growing period is the basis for optimal plant growth and potato yield. Ideal soil fertility and plant nutrition can decrease the severity of A. solani (Lambert et al. 2005; MacDonald et al. 2007).
Under specific conditions, such as drought when plants are unable to get enough nutrients from the soil through the roots, a foliar fertilizer can reduce the nutrient deficiency and reduce plant susceptibility to early blight.
The fertilizer form can also influence the disease progression of A. solani. The use of calcium cyanamide results in a delay of early blight disease, as the fungicidal side effects of degradation products of calcium cyanamide can reduce the initial inoculum in the soil (Volz et al. 2013).
Resistant cultivars
Cultivars with reduced susceptibility to early blight are available; however, no completely resistant genotypes have been found so far. The observed field resistance of varieties to foliage infection is associated with plant maturity. Early maturing cultivars are in general more susceptible, and late maturing cultivars are more resistant to A. solani (Johanson and Thurston 1990; Abuley et al. 2017).
There is no correlation between maturity group and the occurrence of the first early blight symptoms on the leaves, but a strong correlation between maturity group and disease progression. The epidemic in early maturing cultivars starts earlier.
Interestingly, there are varieties within a maturity group which are more resistant to early blight (Johanson and Thurston 1990; Leiminger and Hausladen 2014). Overall, there is a possibility of influencing disease progression by planting more resistant cultivars.
Use of fungicides
The most common method for controlling early blight in potatoes is the use of chemical pesticides. Some fungicides which are used for the control of late blight (Phytophthora infestans) also have some effect on Alternaria solani (e.g. maneb, mancozeb, chlorothalonil, triphenyl tin hydroxide). The most effective fungicides for control of early blight contain active ingredients from the strobilurins group, and azols.
Strobilurins, also known as Quinone outside inhibitors (QoIs), are an important class of fungicides in agriculture because they have a broad-spectrum activity. They inhibit mitochondrial respiration in fungi by binding to the Qo site of the cytochrome b (cytb) complex, blocking electron transfer and inhibiting ATP synthesis (Bartlett et al. 2002).
Carboxamides (SDHI) inhibit the enzyme succinate dehydrogenase (Sdh), a component of complex II in the mitochondrial electron transport chain (Kuhn 1984). Despite the two groups of fungicides having a similar mode of action, SDHI and QoI fungicides show no cross-resistance.
The triazol group belongs to the DMI fungicides, which inhibit one specific enzyme, C14-demethylase. This enzyme plays an important role in ergosterol biosynthesis. Ergosterol is important for functional membrane structure and for the development of functional cell walls.
Due to the single site mode of action of strobilurins (QoI) and carboxamides (SDHI), these fungicides have a high risk of development of resistance. In several potato-growing areas, mutants are found which show a reduced sensitivity in in vitro and in vivo trials and also a reduced efficacy in the field (see Pathogen populations).
Most of these fungicides have a very limited curative activity and should be used preventively. EB control is mainly achieved by multiple and frequent application of protectant fungicides. The optimization of fungicide usage for the control of early blight is a considerable challenge.
The fungus produces huge amounts of secondary inoculum during the growing season. Therefore different DSS (decision support systems) are available to optimize the use of fungicide applications for the management of early blight in potato.
One possibility is to use threshold values based on the disease progress (Leiminger and Hausladen 2012). In some countries, disease management is based on interactive computer-based systems dealing with forecasting favorable weather conditions for infection by Alternaria solani or temperature degree-day thresholds.
Alternatively, the recommendation for the use of fungicides is based on plant development (plant size, plant age, onset of potato flowering).
Overall, the combination of plant age and host resistance, disease progress, and calculated weather-based infection risk is used as the basis for an integrated pest management.
Looking Forward
The control of early blight will be a considerable challenge in the future. The increasing importance of early blight in potatoes is due to a number of factors. Climatic change and global warming will result in more conducive conditions for the infection, growth, and disease progress of the fungus in several potato-growing areas.
Increasing temperatures during the growing season result in favorable conditions for the pathogen, due to its requirement for higher temperatures. More abiotic stress (drought) increases the susceptibility of the plant, and will therefore also promote disease progress.
In addition, integrated pest management based on continuous pesticide treatments will become more and more ineffective. The occurrence of mutants towards different modes of action will result in reduced efficiency in the control of EB by fungicides.
Therefore in the future, successful control of EB requires the implementation of cultural practices, the use of pesticides with a focus on fungicide resistance development, and the cultivation of less susceptible cultivars.