Acrylamide in Food; Scientific Opinion provided by the European Food Safety Authority (EFSA)
Acrylamide in Food; Scientific Opinion provided by the European Food Safety Authority (EFSA)
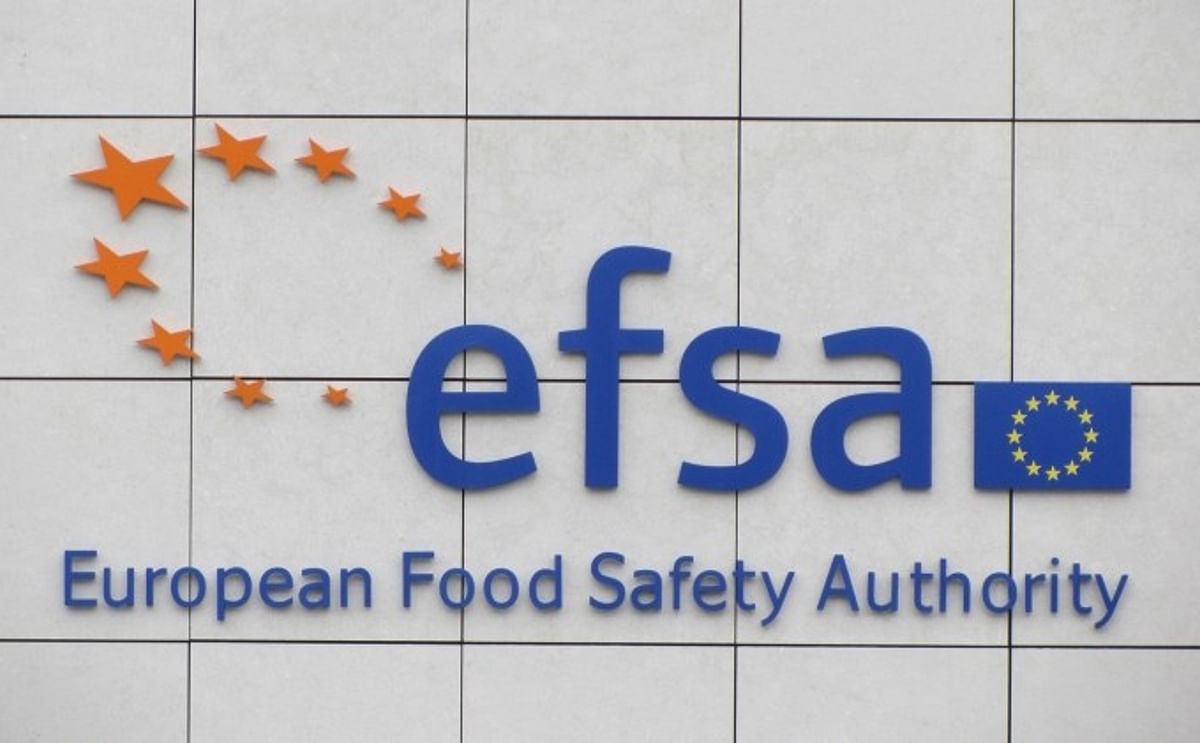
The European Food Safety Authority (EFSA) was asked to deliver a scientific opinion on acrylamide (AA) in food.
AA has widespread uses as an industrial chemical. It is also formed when certain foods are prepared at temperatures above 120 °C and low moisture, especially in foods containing asparagine and reducing sugars.
The CONTAM Panel evaluated 43 419 analytical results from food commodities.
AA was found at the highest levels in solid coffee substitutes and coffee, and in potato fried products.
Mean and 95th percentile dietary AA exposures across surveys and age groups were estimated at 0.4 to 1.9 µg/kg body weight (b.w.) per day and 0.6 to 3.4 µg/kg b.w. per day, respectively.
The main contributor to total dietary exposure was generally the category ‘Potato fried products (except potato crisps and snacks)’.
Preferences in home-cooking can have a substantial impact on human dietary AA exposure.
Upon oral intake, AA is absorbed from the gastrointestinal tract and distributed to all organs. AA is extensively metabolised, mostly by conjugation with glutathione but also by epoxidation to glycidamide (GA).
Formation of GA is considered to represent the route underlying the genotoxicity and carcinogenicity of AA. Neurotoxicity, adverse effects on male reproduction, developmental toxicity and carcinogenicity were identified as possible critical endpoints for AA toxicity from experimental animal studies.
The data from human studies were inadequate for dose-response assessment. The CONTAM Panel selected BMDL10 values of 0.43 mg/kg b.w. per day for peripheral neuropathy in rats and of 0.17 mg/kg b.w. per day for neoplastic effects in mice. The Panel concluded that the current levels of dietary exposure to AA are not of concern with respect to non-neoplastic effects.
However, although the epidemiological associations have not demonstrated AA to be a human carcinogen, the margins of exposure (MOEs) indicate a concern for neoplastic effects based on animal evidence.
Executive Summary
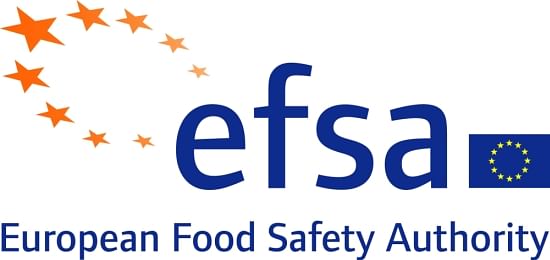
Following a request from the European Commission, the Panel on Contaminants in the Food Chain (CONTAM Panel) was asked to deliver a scientific opinion on acrylamide (AA) in food. The Panel developed the draft scientific opinion which underwent a public consultation from 1 July 2014 to 15 September 2014. The comments received and how they were taken into account when finalising the scientific opinion were published in an EFSA Technical Report (EFSA, 2015).
Read the full report (321 pages; 3.8 Mb pdf)
AA is a low molecular weight, highly water soluble, organic compound. It is used inter alia as an industrial chemical and in the production of polyacrylamides. Heightened concerns about exposure to AA arose in 2002 when it was discovered that it forms when certain foods are prepared at temperatures usually above 120 °C and low moisture. It forms, at least in part, due to a Maillard reaction between certain amino acids, such as asparagine, and reducing sugars. However, several other pathways and precursors have also been proposed to contribute to AA formation. AA forms in numerous baked or fried carbohydrate-rich foods, including French fries, potato crisps, breads, biscuits and coffee. AA is also known to be present in cigarette smoke.
The analytical determination of AA in food products is most frequently performed by high performance liquid chromatographic (HPLC) or gas chromatographic (GC) separation methods with mass spectrometric detection (MS), either in selected ion monitoring (SIM) mode or by tandem mass spectrometry (MS/MS) in multiple reaction mode (MRM) using isotope labelled standards.
In its exposure assessment, the CONTAM Panel evaluated a total of 43 419 analytical results from food commodities collected and analysed since 2010 and reported by 24 European countries and six food associations. Data provided by European countries and those provided by food associations gave overall consistent and complementary information. AA was found at the highest levels in ‘Coffee substitutes (dry)’ (average medium bound (MB) levels of 1 499 µg/kg) and ‘Coffee (dry)’ (average medium bound (MB) levels of 522 µg/kg). However, due to dilution effects, lower levels are expected in ‘Coffee beverages’ and ‘Coffee substitutes beverage’ as consumed by the population. High levels were also found in ‘Potato crisps and snacks’ (average MB level of 389 µg/kg) and ‘Potato fried products (except potato crisps and snacks)’ (average MB level of 308 µg/kg). Lower AA levels were found in ‘Processed cereal-based baby foods’ (average MB level of 73 µg/kg), ‘Soft bread’ (average MB level of 42 µg/kg) and ‘Baby foods, other than cereal-based’ (average MB level of 24 µg/kg). The CONTAM Panel explored the possibility of performing a temporal trend analysis of the AA concentrations in certain foodstuffs across Europe on the basis of the data submitted to EFSA by the European countries. Because of gaps in the databases and the fact that results for different years are not always comparable, a reliable Europe-wide temporal trend analysis is not feasible. However, a dataset of manufacturers’ measurements of AA levels in 40 455 samples of fresh sliced potato crisps from 20 European countries for the years 2002 to 2011 showed a substantial downward trend for mean levels of AA, from 763 ± 91.1 µg/kg in 2002 to 358 ± 2.5 µg/kg in 2011. For other food categories, a similar downward trend was not observed.
Estimation of human exposure to AA revealed that infants, toddlers and other children were the most exposed groups. Depending on the survey and age group, chronic dietary exposure of children was estimated to be on average between 0.5 and 1.9 µg/kg b.w. per day and the 95th percentile was between 1.4 and 3.4 µg/kg b.w. per day. Chronic dietary exposure of adolescents, adults, elderly and very elderly was estimated to be on average between 0.4 and 0.9 µg/kg b.w. per day and the 95th percentile was between 0.6 and 2.0 µg/kg b.w. per day depending on the survey and age group.
The main contributor to the total AA exposure of infants was ‘Baby foods, other than processed cereal-based’ followed by ‘Other products based on potatoes’ and ‘Processed cereal-based baby foods’. The main contributor to the total exposure of toddlers, other children and adolescents was ‘Potato fried products (except potato crisps and snacks)’ representing up to half the total exposure, followed by ‘Soft bread’, ‘Breakfast cereals’, ‘Biscuits, crackers, crisp bread’, ‘Other products based on cereals’ and ‘Other products based on potatoes’. These foods groups were also the main contributors to the total exposure of adults, elderly and very elderly together with ‘Coffee’.
Scenarios were designed in order to assess the influence of specific behaviours (preference for particular products, places of consumption, home-cooking habits) on the total dietary exposure to AA. Scenarios on the preference for particular potato crisps and coffee products resulted in a variation of less than 4 % and 14 %, respectively, of the total dietary exposure to AA. In scenarios on home-cooking behaviours, degree of bread toasting resulted in variations of less than 8 %, while for conditions of potato frying the total dietary exposure to AA could be increased up to 80 %.
In both experimental animals and humans upon oral intake, AA that is not covalently bound to components of the food matrix is extensively absorbed from the gastrointestinal tract. After reaching the systemic circulation, AA is rapidly distributed into the tissues. AA is also able to cross the placenta and is transferred to a small extent into human milk. AA is extensively metabolised, mostly by conjugation with glutathione (GSH) (primarily mediated by glutathione-S-transferases) but also by epoxidation to glycidamide (GA), which is widely distributed into tissues. The formation of GA represents a metabolic activation pathway preferentially mediated by CYP2E1. Mice are more proficient in converting AA into GA than either rats or humans.
Formation of GA is considered to represent the route underlying the genotoxicity and carcinogenicity of AA. Covalent DNA adducts of GA have been amply demonstrated in in vitro models and experimental animals, and these have been used as biomarkers of AA exposure. The N7-guanine adduct derived from GA (N7-GA-Gua) is the most abundant DNA adduct following AA exposure. GA-DNA adducts in experimental animals are found at similar levels in various tissues of the body, although CYP2E1 is primarily located in the liver.
Covalent adducts of AA with DNA have been generated in chemical reactions, but have never been detected in vivo or in vitro in animal or human tissues.
Detoxification of both AA and GA can proceed through conjugation with GSH and the GSH adducts are subsequently converted to mercapturic acids, which are excreted in urine. The mercapturic acids of AA and GA represent the major metabolites and their urinary excretion levels can be used as biomarkers of AA exposure. AA and GA can also react with proteins to form covalent adducts, e.g. with haemoglobin (Hb). Also these Hb adducts represent important biomarkers of AA exposure.
Several studies have reported various approaches to physiologically based pharmacokinetic (PBPK) modelling of AA absorption, metabolism, and disposition with the goal of predicting human internal exposures to AA and GA (i.e. area under the curve, AUC) for use in reducing the uncertainty in risk assessment inherent in animal to human extrapolations.
PBPK models allow derivation of human-equivalent doses (HEDs), which could be used to convert external doses of AA that produce critical effects in animal studies to the human external doses required to produce equivalent AUC values for either AA or GA, depending on the toxic endpoint used. The HEDs derived from equivalent AA-AUCs in rats and mice suggest that endpoints related to AA-mediated effects (e.g. neurotoxicity) require 4- to 6-fold higher doses in rats when compared to humans, based on inter-species differences in toxicokinetics. However, 0.5- to 0.7-fold lower doses of AA would be required in mice to produce equivalent GA-AUCs for genotoxicity-related endpoints when compared to humans.
Toxicological studies with AA have been conducted in rats, mice, monkeys, cats and dogs, using various dosing protocols and routes of exposure. Oral LD50 values for AA were reported to be > 150 mg/kg b.w. for rats, 107 mg/kg b.w. for mice, and 150–180 mg/kg b.w. for rabbits and guinea pigs.
Adverse effects reported in repeated dose toxicity studies of AA in rats, mice, monkeys, cats and dogs consisted of loss of body weight and effects on the nervous system reflected by hind-limb paralysis, reduction in rotorod performance and/or histopathological changes in peripheral nerves and nervous system structures. In mice, effects reported in addition to the neurotoxicity consisted of effects on the testes, including the degeneration of epithelia in spermatids and spermatocytes, the reduction of spermatozoa, and the presence of multinucleate giant cells, as well as forestomach hyperplasia, hematopoietic cell proliferation of the spleen and preputial gland inflammation, lung alveolar epithelium hyperplasia and cataract and for female mice ovarian cysts.
In rats, effects reported in addition to the neurotoxicity, included atrophy of skeletal muscle, testicular atrophy, distended urinary bladders, increased prevalence of duct ectasia in preputial glands, hematopoietic cell proliferation in the spleen, bone marrow hyperplasia, ovarian atrophy, degeneration of the retina, exfoliated germ cells epididymis, hepatocyte degeneration and liver necrosis, bone marrow hyperplasia, mesenteric lymph node cellular infiltration and pituitary gland hyperplasia.
Thirteen-week and 2-year studies in mice and rats dosed with GA revealed adverse effects that were generally similar to those reported for AA. Rats were more sensitive to the neurotoxic effects of AA and GA than mice, and neurotoxicity in rats (such as hind-leg paralysis and peripheral neuropathy) was consistently associated with lower AA doses and greater severity when compared to equimolar concentrations of GA.
Rodent studies have demonstrated adverse effects of AA on male reproductive parameters including reduced sperm counts and effects on sperm and testis morphology with a no-observed-adverse-effect level (NOAEL) of approximately 2 mg/kg b.w. per day.
Rat and mouse studies have shown some signs of developmental toxicity (increased incidence of skeletal variations, slightly impaired body weight gain, histological changes in the central nervous system, and neurobehavioural effects) at exposure levels that in some cases are also associated with maternal toxicity. The lowest NOAEL reported for developmental toxicity was 1.0 mg/kg b.w. per day from studies in rats exposed gestationally and neonatally.
The genotoxicity of AA, as well as of its reactive metabolite GA, has been extensively studied. In vitro genotoxicity studies indicate that AA is a weak mutagen in mammalian cells but an effective clastogen. GA is a strong mutagen and a clastogen. It induces mutations via a DNA adduct mechanism. In vivo, AA is clearly genotoxic in somatic and germ cells. AA exerts its mutagenicity via metabolism by CYP2E1 to GA. AA can also induce gene mutations by a pathway involving the generation of reactive oxygen species (ROS) and oxidative DNA damage.
AA is carcinogenic in multiple tissues in both male and female mice and rats. In rats, the major tumours produced by AA are adenomas, fibroadenomas and fibromas of the mammary gland, thyroid gland follicular cell adenomas or carcinomas, and in F344 rats, testes or epididymis tunica vaginalis mesotheliomas. In mice, the major tumours produced by AA are: Harderian gland adenomas, mammary gland adenoacanthomas and adenocarcinomas, lung alveolar and bronchiolar adenomas, benign ovary granulosa cell tumours, skin sarcomas, and stomach and forestomach squamous cell papillomas in females, and Harderian gland adenomas and adenocarcinomas, lung alveolar and bronchiolar adenomas and carcinomas, and stomach squamous papillomas and carcinomas in males.
A similar spectrum of tumours is observed when equimolar concentrations of GA were administered in drinking water to rats and mice, which is consistent with GA being the proximate carcinogenic metabolite of AA.
AA is an electrophilic molecule which can undergo Michael addition-type reactions with nucleophilic target molecules. In particular, activated thiolate moieties in cysteine residues of enzymes and other functional proteins, e.g. in neuronal cells or spermatocytes, have been described as targets. The neurotoxic properties of AA are considered to originate mainly from this type of reactivity.
AA shows some reactivity towards nucleic acids, whereas reports on the formation of DNA adducts in vivo suggest that GA is mainly, if not exclusively, responsible for the formation of DNA adducts in AA-treated animals.
Evidence from the available studies in the literature on hormonal and endocrine effects of AA is equivocal. This is particularly true for changes in hormone levels in AA-treated animals which were reported in some studies. Mechanistic hypotheses on local endocrine effects of AA which may explain tumour formation in certain hormone or paracrine-regulated target tissues lack experimental proof.
There is a wide range of epidemiological studies investigating possible effects of AA in humans. Two cohort studies considered occupational exposure to AA and did not indicate an increased cancer risk. Associations between AA exposure through diet and cancer risk have been analysed in at least 36 publications, based on 16 epidemiological studies considering several cancer sites.
For most cancers there is no consistent indication for an association between AA exposure and increased risk. A few studies suggested an increased risk for renal cell, and endometrial (in particular in never-smokers) and ovarian cancer, but the evidence is limited and inconsistent. Moreover, one study suggested a lower survival in non-smoking women with breast cancer with a high pre-diagnostic exposure to AA but more studies are necessary to confirm this result.
Two studies reported an inverse relation between AA exposure (measured by levels of AA and GA adducts) and birth weight and other markers of fetal growth. The CONTAM Panel noted that it has not been established whether the association between dietary AA exposure and these outcomes is causal.
Studies among workers occupationally exposed to AA showed an increased risk of neurological alterations, including mostly the peripheral, but also the central nervous system.
From all data available, the CONTAM Panel identified four possible critical endpoints for AA toxicity, i.e. neurotoxicity, effects on male reproduction, developmental toxicity, and carcinogenicity.
The data from human studies were not adequate for dose-response assessment. Therefore, the CONTAM Panel considered the data from studies on experimental animals to establish the reference points. The CONTAM Panel performed benchmark dose (BMD) analyses on data for neurotoxicity and on the tumour incidences induced by AA in experimental animals. The CONTAM Panel selected the value of 0.43 mg/kg b.w. per day derived as the lowest BMDL10 from the data on incidences of peripheral nerve (sciatic) axonal degeneration in male F344 rats exposed to AA in drinking water for two years as the reference point for non-neoplastic effects. Based on the fact that this BMDL10 is lower than the NOAEL of approximately 2 mg/kg b.w. per day for adverse effects on male reproductive parameters and of 1.0 mg/kg b.w. per day for developmental toxicity, the CONTAM Panel concluded that using the BMDL10 for neurotoxicity as the reference point is conservative when considering possible non-neoplastic effects of AA.
For neoplastic effects, the CONTAM Panel selected as a reference point the value of 0.17 mg/kg b.w. per day derived as the lowest BMDL10 from data on incidences of Harderian gland adenomas and adenocarcinomas in male B6C3F1 mice exposed to AA for two years. The CONTAM Panel noted that the Harderian gland is an organ absent in humans, but that in rodents this organ is a sensitive target tissue to detect compounds that are both genotoxic and carcinogenic. Taking into account that target tissues for tumour formation by a given genotoxic carcinogen may differ between rodents and humans, the CONTAM Panel considered the most sensitive target tissue in rodent bioassays, the Harderian gland, a conservative endpoint for assessment of the risk for neoplastic effects of AA in humans.
The fact that AA and its metabolite GA are positive in a variety of genotoxicity tests indicates that AA is of concern with respect to genotoxicity. Therefore, the CONTAM Panel considered it inappropriate to establish a tolerable daily intake (TDI).
Risk characterisation for non-neoplastic effects was performed using the margin of exposure (MOE) approach and the BMDL10 value of 0.43 mg/kg b.w. per day for the most relevant and sensitive endpoint for neurotoxicity, i.e. the incidence of peripheral nerve (sciatic) axonal degeneration observed in F344 rats exposed to AA in drinking water for two years in the NTP study. MOE values for the neurotoxic effects ranged from 1 075 (minimum LB) to 226 (maximum UB) for the mean exposure, and from 717 (minimum LB) to 126 (maximum UB) for the 95th percentile exposure estimates across surveys and age groups). Taking into account differences between species and within the human population, the Panel concluded that the MOEs across surveys and age groups are not of concern. However, the Panel noted that the MOEs for the 95th percentile UB exposure estimates for toddlers and other children are close to the value that might be of concern for neurotoxicity.
For the risk characterisation for neoplastic effects, the MOE approach for compounds that are both genotoxic and carcinogenic is considered appropriate, using as the reference point the BMDL10 of 0.17 mg/kg b.w. per day, i.e. the lowest BMDL10 from data on incidences of Harderian gland adenomas and adenocarcinomas in male B6C3F1 mice exposed to AA for two years in the NTP study. Comparison of the data on human exposure levels to AA across surveys and age groups reported above to this BMDL10 of 0.17 mg/kg b.w. per day, reveals MOE values that range from 425 (minimum LB) to 89 (maximum UB) for the mean exposure estimates, and from 283 (minimum LB) to 50 (maximum UB) for the 95th percentile exposure estimates across all surveys and age groups. The EFSA Scientific Committee concluded that, for substances that are both genotoxic and carcinogenic, an MOE of 10 000 or higher, based on a BMDL10 from an animal study, and taking into account overall uncertainties in the interpretation, would be of low concern from a public health point of view. Since the MOEs calculated are all substantially lower than the value of 10 000, the CONTAM Panel concluded that, although the available human studies have not demonstrated AA to be a human carcinogen, the MOEs across surveys and age groups indicate a concern with respect to neoplastic effects.
The CONTAM Panel noted that AA is a germ cell mutagen and that there are at present no established procedures for risk assessment using this endpoint.
Finally, the CONTAM Panel makes the following recommendations: the reporting of AA occurrence data should be improved regarding the mode of preparation of the products before analysis. Duplicate diet studies are recommended in order to improve exposure assessment, since they provide a more accurate indication of AA levels in food as prepared and consumed at home. Data on urinary metabolites levels from individuals participating in the duplicate diet studies should be generated for the purpose of validation of the biomarkers. An up-to-date OECD compliant extended one-generation or two-generation reproductive toxicity study investigating the effects of AA on sperm parameters and including a detailed histopathological examination of the testis and accessory glands as well as investigating the effects on development until puberty should be conducted. If further epidemiological studies are conducted to assess possible associations between dietary AA intake and risk of cancers (e.g. endometrium, ovary and renal cells), they should have improved measurement of AA exposure, and should be sufficiently powered. Further epidemiological studies are required to confirm or refute the inverse relation between dietary AA intake and birth weight and other markers of fetal growth observed in two studies. Improved approaches for the detection and risk assessment of germ cell mutagens should be developed, and applied to AA and GA.
Read the full report (321 pages; 3.8 Mb pdf)